Physics’ Kottos Develops an Innovative Power Limiter
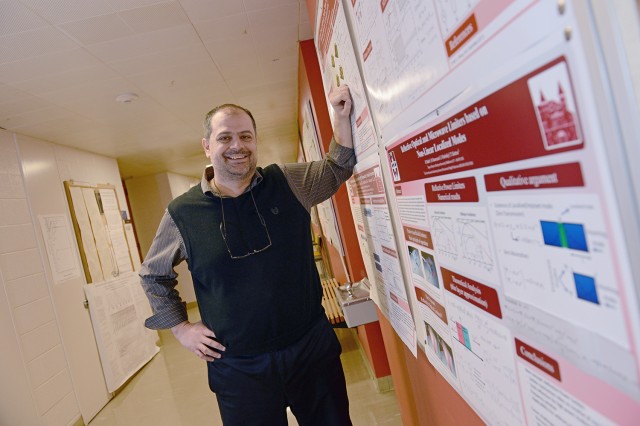
The U.S. Air Force has taken a keen interest in the recent work of Tsampikos Kottos, the Douglas J. and Midge Bowen Bennet Associate Professor of Physics. Kottos, along with Graduate Research Assistant Eleana Makri, Hamidreza Ramezani Ph.D. ’13 (now a postdoc at U.C. Berkeley) and Dr. Ilya Vitebskiy (AFRL/Ohio), has come up with a theoretical way to build a more effective, reusable power limiter.
Generally speaking, the function of a power limiter is to protect a sensor — be it the human eye, an antenna, or other sensitive equipment — from high-intensity radiation, like that generated by high-power lasers.
Kottos, Makri, Ramezani and Vitebskiy published a paper titled “Non-Linear Localized Modes Give Rise to a Reflective Optical Limiter” [Phys. Rev. A 89, 031802(R) (2014)] that was highlighted in Washington, D.C. at the spring review meeting of the Air Force Office of Scientific Research (AFOSR) as one of the main research achievements in electromagnetics of 2014 that can potentially benefit the U.S. Air Force. Now, with the Air Force’s help, Kottos is taking the necessary steps to make the project become a reality.
Generally speaking, there are two categories of limiters — dynamic and passive. These new limiters are of the passive variety.
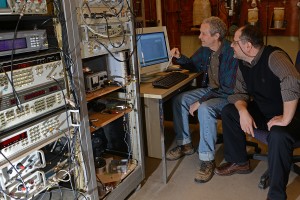
“Dynamic limiters are very slow,” explained Kottos. “They consist of many parts, and then these parts have to communicate with each other. So these are not very good. Passive limiters perform the limiting action — the filtering of the high power — based on the intrinsic properties of the materials.”
So, passive limiters are the way to go.
When striving to produce better passive limiter components, one can synthesize new materials (which Wes is not currently equipped to do on-site), or one can rely on existing materials and try to design or propose geometries that will improve the efficiency of existing materials.
Since the dawn of lasers in the 1960s, the standard filtering protection has been based on the use of what are called sacrificial limiters. When high-intensity light passes through a sacrificial limiter, the materials absorb the energy, heat up and melt, becoming opaque. The light is blocked and the sensor is protected, but the limiter is destroyed and must be swapped out like a burnt lightbulb. This is less than ideal, as it’s expensive and time-consuming to replace.
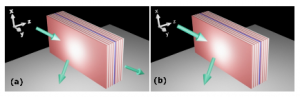
“We want to propose a clever limiter which is not going to sacrifice itself in order to save the sensor on the other side,” Kottos said. “What we are proposing is to create two stacks of alternate layers, A and B. This is what people usually call a Bragg mirror. Such a structure creates a frequency window for which light is completely reflected irrespective of its intensity. This solves one part of the problem but it creates another one. Namely, we want ‘non-harmful,’ low-intensity light to be transmitted. How can we achieve this? Well, the simple way is by creating a ‘bridge.’ But the bridge has to be clever. It must allow low intensity light to pass and block high intensity light. One way to do this is to make sure that the bridge will collapse if high intensity light goes through.”
Kottos’ new work involves placing a defect layer of dissipative nonlinearity (“the bridge”) in the middle of the Bragg mirror. The nonlinear properties of the materials increase dissipation for high light intensities. Strange as it sounds, losses (dissipation) can rescue the limiter (bridge) from high power light and reflect the energy into space.
“To understand this we need to think of how three oscillators coupled with springs — with the middle one having friction (the dissipation layer) — will behave when energy is pumped into the system. Say the left one is excited, displacing it from the equilibrium position. Then energy will move from the left one to the right one via the spring and then will continue to the third via the second spring that connects the last two together. Via this process, some energy will be turned to heat via the friction of the middle oscillator. Now let’s further increase the friction in the middle, which in optics is achieved via the dissipative nonlinear mechanism when incident power is increased. Obviously the process will be repeated, but now more energy will be radiated as heat since the friction in the middle is higher. But what will happen if the friction in the middle is huge, corresponding to high incident power in optics which will trigger high dissipative nonlinearities?”
The intuitive prediction is that friction-generated heat will burn the middle oscillator. But students in Kottos’ “Waves and Oscillations” course would predict that a huge friction will turn the middle oscillator into an immovable wall, neutralizing the friction and reflecting all the energy back without letting it pass to the third oscillator. And this is exactly the mechanism Kottos and co. are exploring, but in the optics realm.
“We knew this principle since centuries ago — it’s called impedance mismatching,” Kottos said. “The more you create an absorber, the more the energy that’s not absorbed but reflected back. I know that’s an oxymoron, but this is how it happens. The reason that we did not use this property up to now is rather psychological. In most cases we strive to ‘match’ things and we are used to this way of thinking. In this specific case we thought the other way around.”
The experimental realizations of these new theoretical optical limiters are currently being investigated at two U.S. labs. With time, the Wes group hopes to continue refining its proposal to further increase the limiters’ effectiveness. A further step down the road is to implement the same idea acoustically.
“I am hopeful that the experimental group of Professor Fred Ellis at Wes will be able to demonstrate the applicability of this idea in acoustics,” said Kottos. “Discussions along this line of research are in progress.”